Most currently used vaccines work by getting the body to produce
antibodies against the disease. Antibodies are unable to attack
the malaria parasite once it has invaded liver cells and thus
the approach of the Malaria Vaccine Trials Group in Oxford has
been to design vaccines that will induce potent T-cell responses
against the liver stage of malaria infection.
T-cells are a type of white blood cell called lymphocytes that
circulate in the blood. This approach could prevent both blood-stage
infection (and thus disease) and also prevent malaria transmission
in endemic areas. The vaccines stimulate populations of T-cells
that will destroy liver cells that are harbouring the malaria
parasite and thus prevent parasite development. The T-cells recognise
the infected liver cells as they express small peptides from malaria
on their surface.

To date we have generated three different types of vaccines, all
of which have been in clinical trials in humans in a variety of
combinations:
1. DNA vaccine: This is a plasmid
of DNA (a small circular piece of DNA) that encodes what we believe
are immunologically important components of the malaria pathogen
- several peptide epitopes that we know are recognised by T-cells
(a so-called multi-epitope string) and a whole protein called
thrombospondin related adhesion protein (TRAP). The whole vaccine
is therefore known as DNA ME-TRAP.
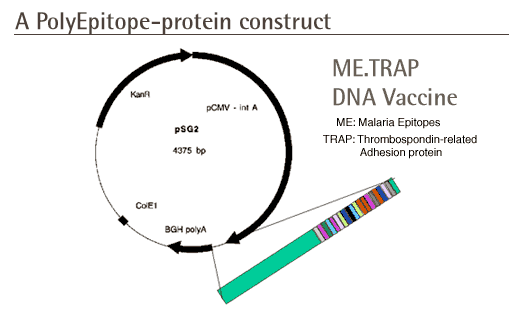
2. MVA: This is an attenuated
virus vaccine called modified vaccinia virus Ankara (MVA). It
is derived from the old smallpox vaccine but has been biologically
altered so that it is unable to replicate in human cells but still
generates a potent immune response. We have genetically altered
this virus so that it encodes the same epitopes and protein as
in the DNA vaccine (i.e. ME-TRAP). We have also genetically engineered
MVA that expresses a different liver stage protein called the
circumsporozoite protein (MVA - CSO)
3. FP9: This is a cousin of MVA and again
has been biologically altered so that it is replication deficient
and cannot cause disease in humans but is still capable of stimulating
an immune response. We have manufactured an FP9 virus that encodes
for ME-TRAP.
4. AdC63: This is a different attenuated vaccine vector, based on an adenovirus that infects chimpanzees. We have made AdC63 expressing ME-TRAP. Again, it cannot cause disease in humans but adenovirus vaccines are very good at inducing immune responses (both T cells and antibodies) against the antigens that they express. However a major limitation is that most people have already been infected by adenoviruses, which cause upper respiratory tract infections, and that blunts immune responses to adenovirus-based vaccines”. This problem of “anti-vector immunity” is even greater in Africa where a malaria vaccine is needed most: the prevalence of antibodies to common adenovirus strains is highest in Sub-Saharan Africa. But chimpanzees have their own set of adenoviruses which appear seldom to infect humans and one of these forms the backbone of the new vaccine, allowing us to use the full power of the adenovirus vector in our vaccine.
Studies are also being conducted in collaboration with GlaxoSmithKline
Biologicals that are financed by the Malaria
Vaccine Initiative. This study will test one of our MVA based
vaccines (MVA-CSO) in combination with the RTS,S/AS02A vaccine
produced by GlaxoSmithKline Biologicals. The RTS,S/AS02A vaccine
has already shown promising results in clinical trials when used
alone and the aim of the collaborative studies is to improve on
this further.
The use of these vaccines alone produces only very modest immune
responses. However, this immune response can be significantly
augmented when one type of vaccine is used to "prime"
the immune system and a second different vaccine (encoding the
same genetic information) is used to "boost" the response.
The response to vaccination is measured by counting T-cells that
secrete a cytokine (a chemical secreted by one cell that acts
on a neighbouring cell) called gamma interferon. The graphs below
show that the sequence of vaccination is critical to optimising
the results i.e. DNA followed by MVA yields better results than
the other way around. The order of vaccination is less important
when using FP9 and MVA.
This enhanced capacity to induce strong effector
T-cells should have a widespread impact in both human and veterinary
medicine. This new vaccine technology can be used to make both
preventative and therapeutic vaccines against infectious diseases
such as TB, HIV and persistent viral hepatitis but also malignancies
such as melanoma and bowel cancer.
|